ليزر الإلكترون الحر
ليزر الإلكترون الحر (بالإنجليزية: Free-electron laser) هو الليزر الذي يَشتركُ في نفس الخصائص البصريةِ كأي ليزر تقليدي مثل إرسال شعاع يَشْملُ الإشعاعِ الكهرومغناطيسيِ المتماسكِ الذي يُمْكِنُ أَنْ يَصلَ إلى قوَّةَ عاليةَ، لكن يجب استخدام بَعْض مبادئِ التشغيل المختلفةِ لتَشكيل الشعاعِ. على خلاف الغازِ، السائل، أَو ليزر الحالة الصلبةِ مثل ليزرِ دايود، الذي تكون فيها الالكترونات مثارة في الحالةِ الذرّيةِ أَو الجزيئيةِ المقيدة، ويستخدم ليزر الإلكترون الحر شعاع إلكتروني نسبى كالوسط الذي يَتحرّكُ بحرية خلال تركيب مغناطيسي، ومن ثم مصطلح الإلكترون الحر. ّ يمتلك ليزر الإلكترون الحُرَّ مدى الترددِ الأوسعِ من أي نوع ليزر آخر، ويُمكنُ أَنْ يَكُونَ على نحو أكثر اتساعا ويَتراوحُ حالياً طوله الموجى مِنْ المايكروويف، خلال إشعاع التتراهيرتزِ والأشعة تحت الحمراءِ، إلى الطيفِ المرئيِ ،و إلى الأشعة فوق البنفسجيِ، وإلى الأشعة السينيةِ.
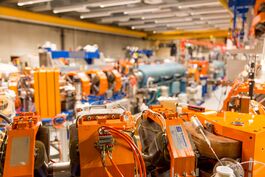
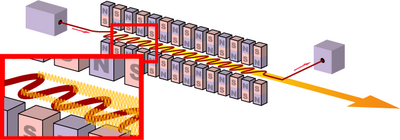
تم اختراع ليزر الإلكترون الحر مِن قِبل جون مادي في عام 1976 في جامعةِ ستانفورد. انطلق العملُ مِنْ بحثِ عملَ مِن قِبل (هانز موتز) الذي اقترحَ الترتيبَ المتمايلَ المغناطيسيَ الموجود في قلبِ ليزر الإلكترون الحُرِّ. استعمل (مادي) عديد من الأشعة إلكترونية متمايلة وبطول 5 أمتاراً لتَضْخيم إشارة. مباشرة بعد ذلك، مختبرات أخرى بالمعجّلاتِ بَدأتْ بتَطوير مثل هذا الليزر
. . . . . . . . . . . . . . . . . . . . . . . . . . . . . . . . . . . . . . . . . . . . . . . . . . . . . . . . . . . . . . . . . . . . . . . . . . . . . . . . . . . . . . . . . . . . . . . . . . . . . . . . . . . . . . . . . . . . . . . . . . . . . . . . . . . . . . . . . . . . . . . . . . . . . . . . . . . . . . . . . . . . . . . .
خلق الشعاع
لتكوين ليزر الإلكترون الحر فان شعاع الالكترونات تزاد سرعته حتى تصل إلى سرعة الضوء تقريبا (تقنيا المعروفة بـــ السرعة النسبية). يمر الشعاع خلال ليزر الإلكترون الحر مُذَبذب على شكل دوري ,ويقطع حقل مغناطيسي، وينتج من ترتيب مغناطيسات مع الأقطاب المتناوبة ضمن تجويف الليزر على طول طريق الشعاع كما يدعى هذا الصف من المغناطيسات أحيانا بــ "المتمايل" لأنه يجبر الالكترونات على اتخاذ شكل جيب الزاوية في طريقها. ينتج عن زيادة سرعة الالكترونات على طول الطريق في إطلاق الفوتون. منذ انبعاث حركةِ الإلكترون في المرحلةِ بحقلِ الضوءِ، اضيفُت الحقولَ سوية بشكل متماسك. منذ انبعاث حركةِ الإلكترون في المرحلةِ بحقلِ الضوءِ، اضيفُت الحقولَ سوية بشكل متماسك.بينما يسبب الإشعاع المتموج الإشعاع المستقل للالكترونات وعدم استقرار في شعاعِ الإلكترون يَنْتجُ مِنْ تفاعلاتِ تذبذباتِ الالكترونات في المتموجات والإشعاع الذي ينبعث يؤدى إلى تكوين حزم من الالكترونات التي توصل الإشعاع في المرحلة إلى بعضهم البعض. يمكن للطول الموجى للضوء المنبعث أن يُنغّمَ بسهولة بتعديل طاقةِ شعاعِ الإلكترون أَو قوّةَ الحقلِ المغناطيس للتمويجات.
The transverse acceleration of the electrons across this path results in the release of photons, which are monochromatic but still incoherent, because the electromagnetic waves from randomly distributed electrons interfere constructively and destructively in time. The resulting radiation power scales linearly with the number of electrons. Mirrors at each end of the undulator create an optical cavity, causing the radiation to form standing waves, or alternately an external excitation laser is provided. The radiation becomes sufficiently strong that the transverse electric field of the radiation beam interacts with the transverse electron current created by the sinusoidal wiggling motion, causing some electrons to gain and others to lose energy to the optical field via the ponderomotive force.
This energy modulation evolves into electron density (current) modulations with a period of one optical wavelength. The electrons are thus longitudinally clumped into microbunches, separated by one optical wavelength along the axis. Whereas an undulator alone would cause the electrons to radiate independently (incoherently), the radiation emitted by the bunched electrons is in phase, and the fields add together coherently.
The radiation intensity grows, causing additional microbunching of the electrons, which continue to radiate in phase with each other.[1] This process continues until the electrons are completely microbunched and the radiation reaches a saturated power several orders of magnitude higher than that of the undulator radiation.
The wavelength of the radiation emitted can be readily tuned by adjusting the energy of the electron beam or the magnetic-field strength of the undulators.
FELs are relativistic machines. The wavelength of the emitted radiation, , is given by[2]
or when the wiggler strength parameter K, discussed below, is small
where is the undulator wavelength (the spatial period of the magnetic field), is the relativistic Lorentz factor and the proportionality constant depends on the undulator geometry and is of the order of 1.
This formula can be understood as a combination of two relativistic effects. Imagine you are sitting on an electron passing through the undulator. Due to Lorentz contraction the undulator is shortened by a factor and the electron experiences much shorter undulator wavelength . However, the radiation emitted at this wavelength is observed in the laboratory frame of reference and the relativistic Doppler effect brings the second factor to the above formula. In an X-ray FEL the typical undulator wavelength of 1 cm is transformed to X-ray wavelengths on the order of 1 nm by ≈ 2000, i.e. the electrons have to travel with the speed of 0.9999998c.
متغير ويگلر للقوة K
K, a dimensionless parameter, defines the wiggler strength as the relationship between the length of a period and the radius of bend,[بحاجة لمصدر]
where is the bending radius, is the applied magnetic field, is the electron mass, and is the elementary charge.
Expressed in practical units, the dimensionless undulator parameter is .
الآثار الكمومية
In most cases, the theory of classical electromagnetism adequately accounts for the behavior of free electron lasers.[3] For sufficiently short wavelengths, quantum effects of electron recoil and shot noise may have to be considered.[4]
الإنشاء
المعجلات
اليوم, يَتطلّبُ ليزرَ الإلكترون الحُرِّ استعمال معجّلِ إلكترون بحِمايته المرتبطةِ، كما عُجّلَ الإلكترونات خطر إشعاعِ. هذه المعجّلاتِ تُشغّلُ نموذجياً مِن قِبل (الكليسترونات)، التي تَتطلّبُ تجهيز فرق جهد عاليِ. عادة، شعاع الإلكترون يجب أنْ يُبقي في الفراغ الذي يَتطلّبُ استعمال المضخاتِ العديدةِ على طول طريقِ الشعاعَ. بينما هذه الأجهزةِ ضخمةُ وغاليةُ،كما يُمْكِنُ لليزر الإلكترون الحُرِّ أَنْ يُنجزَ سلطاتَ بالغة الذروةَ وعاليةَ جداً، والأنواع المختلفة مِنْ ليزارات الالكترونات الحرة يَجْعلُهم مطلوبين جداً في عِدّة مجالات، تتضمن التشخيصِ الطبيِ والاختبار غيرِ التدميريِ.
Infrared and terahertz FELs
The Fritz Haber Institute in Berlin completed a mid-infrared and terahertz FEL in 2013.[5][6]
الأشعة السينية في ليزر الالكترونات الحرة
قلة المرايا المناسبةِ في فوق البنفسجيةِ الشديدة وأنظمةِ الأشعة السينيةِ يَمْنعانِ عمليةَ مُذَبذِبِ ليزر الإلكترون الحر ؛ ولذلك، لابدّ أن يكون هناك تكبير مناسب على طريق فردى مِنْ شعاعِ الإلكترون خلال تموج لجَعْل الليزر نافع. ويَستعملُ ليزرُ الأشعة الإلكترونية الحرة السينية تمويجات طويلة. المبدأ الأساسي للنبضاتِ الشديدة مِنْ ليزرِ الأشعة السينيةَ يَكْمنُ في مبدأِ الإشعاعِ المُضَخَّمِ الذاتيِ التلقائيِ (إس أي إس إي)، الذي يُؤدّي إلى حزم من الإلكترونات. بداية كُلّ الإلكترونات مُوَزَّعة بانتظام وهم يَبْعثونَ إشعاعَ تلقائيَ متفكّكَ فقط. خلال تفاعلِ هذا الإشعاعِ وتذبذباتِ الإلكترونات، يَنجرفونَ إلى حزم ضوئية مفصولة مِن قِبل نظير مسافةِ إلى طولِ موجة إشعاعِ واحد. خلال هذا التفاعلِ، كُلّ الإلكترونات تَبْدأُ ببَعْث إشعاعِ متماسكِ في هذا الطور. بكلمة أخرى، كُلّ الإشعاع المَبْعُوث يُمْكِنُ أَنْ يُعزّزَ نفسه بشكل مثالي حيث موجة تُتوّجُ ومنخفضات موجةِ تُركّبُ دائماً على أحدهما الآخر في أفضل طريقِ محتملِ. هذا يُؤدّي إلى زيادةِ طبيعية مِنْ قوَّةِ الإشعاعِ المَبْعُوثةِ، ويُؤدّي إلى كثافةِ الشعاعِ العاليةِ أمثلة لتشغيل الوسائلِ على مبدأِ (إس إي إس إي) ليزر الالكترونات الحرة يَتضمّنانِ:- 1- ليزرَ الإلكترون الحُرَّ في هامبورغ، 2- مصدر ضوء (ليناك) المتماسك (إل سي إل إي) في مختبرِ معجّلِ (إس إل أي سي) الوطني، 3- ليزر إلكترون الأشعة السينيةِ الحُرِّ الأوروبيِ، توجد مشكلة واحدة مَع ليزر الالكترونات الحرة (إس أي إس إي) هي قلةُ التماسكِ المؤقت بسبب مشاكل بداية العملية. لتَفادي هذا، يُمْكِنُ أَنْ يتم توزيع ليزر الالكترونات الحرة مَع ليزر آخر منغّمَ إلى رنينِ ليزر الالكترونات الحرة مثل هذه البذرةِ المتماسكةِ بشكل دنيوي يُمْكِنُ أَنْ تُنتَجَ بالوسائلِ الأكثرِ تقليدية، مثل بالجيلِ العاليِ المتناسقِ (إتش إتش جي) يَستعملُ نبضةَ ليزرِ بصريةِ. هذا يُؤدّي إلى التكبيرِ المتماسكِ مِنْ الإشارةِ الداخلة؛ في الواقع، جودة الليزرِ الناتجَ مُمَيَّزةُ بما داخله. بينما (إتش إتش جي) متوفرة في أطوالِ موجية أقل من الموجات فوق البنفسجيةِ المتطرّفةِ، كما يعتبر البَذْر حالياً لَيسَ عمليَ في أطوالِ موجة الأشعة السينيةِ بسبب قلةِ ليزرِ الأشعة السينيةِ التقليديِ.
Examples of facilities operating on the SASE FEL principle include the:
- Free electron LASer in Hamburg (FLASH)
- Linac Coherent Light Source (LCLS) at the SLAC National Accelerator Laboratory
- European x-ray free electron laser (EuXFEL) in Hamburg[7]
- SPring-8 Compact SASE Source (SCSS) in Japan
- SwissFEL at the Paul Scherrer Institute in Switzerland
- SACLA at the RIKEN Harima Institute in Japan
- PAL-XFEL (Pohang Accelerator Laboratory X-ray Free-Electron Laser) in Korea
In 2022, an upgrade to Stanford University’s Linac Coherent Light Source (LCLS-II) used temperatures around −271 °C to produce 106 pulses/second of near light-speed electrons, using superconducting niobium cavities.[8]
Seeding and Self-seeding
One problem with SASE FELs is the lack of temporal coherence due to a noisy startup process. To avoid this, one can "seed" an FEL with a laser tuned to the resonance of the FEL. Such a temporally coherent seed can be produced by more conventional means, such as by high harmonic generation (HHG) using an optical laser pulse. This results in coherent amplification of the input signal; in effect, the output laser quality is characterized by the seed. While HHG seeds are available at wavelengths down to the extreme ultraviolet, seeding is not feasible at x-ray wavelengths due to the lack of conventional x-ray lasers.
In late 2010, in Italy, the seeded-FEL source FERMI@Elettra[9] started commissioning, at the Trieste Synchrotron Laboratory. FERMI@Elettra is a single-pass FEL user-facility covering the wavelength range from 100 nm (12 eV) to 10 nm (124 eV), located next to the third-generation synchrotron radiation facility ELETTRA in Trieste, Italy.
In 2001, at Brookhaven national laboratory, a seeding technique called "High-Gain Harmonic-Generation" that works to X-ray wavelength has been developed.[10] The technique, which can be multiple-staged in an FEL to achieve increasingly shorter wavelengths, utilizes a longitudinal shift of the radiation relative to the electron bunch to avoid the reduced beam quality caused by a previous stage. This longitudinal staging along the beam is called "Fresh-Bunch".[11] This technique was demonstrated at x-ray wavelength[12][13] at Trieste Synchrotron Laboratory.
A similar staging approach, named "Fresh-Slice", was demonstrated at the Paul Scherrer Institut, also at X-ray wavelengths. In the Fresh Slice the short X-ray pulse produced at the first stage is moved to a fresh part of the electron bunch by a transverse tilt of the bunch.[14]
In 2012, scientists working on the LCLS found an alternative solution to the seeding limitation for x-ray wavelengths by self-seeding the laser with its own beam after being filtered through a diamond monochromator. The resulting intensity and monochromaticity of the beam were unprecedented and allowed new experiments to be conducted involving manipulating atoms and imaging molecules. Other labs around the world are incorporating the technique into their equipment.[15][16]
الأبحاث
التطبيقات الطبية
بحث مِن قِبل الدّكتورِ جلين إدواردز والزملاءِ في مركزِ (إف إي إل) بجامعة فاندربيلت في عام 1994 وَجدوا تلك الأنسجةِ الناعمةِ مثل جلدِ، قرنية، ونسيج المخ يُمْكِنُ أَنْ يُقْطَعَ، أَو يُستأصلَ، باستعمالُ ليزر الإلكترون الحر ذات أطوالَ موجة حول 6.45 مايكرو متر بأقل ضرر ناتج للأنسجة المحيطة. هذا قادَ إلى أبحاث ذات مدى ابعد وفي النهاية جراحاتِ على البشرِ، في أول استعمال ليزر إلكترون حُرِّ.وكانت البداية في 1999، واستخدم الدكتور (مايكل كوبلياند) والدكتور (بيتر كونارد) تأسيس (كيك) بادخار غُرَفَ تشغيل ليزر الالكترونات الحرة في مركزِ (فاندربيلت إف إي إل) وقاموا بأداء ثلاث جراحات التي فيها استأصلوا أورام الورم السحائي الدماغية. بدأت الدّكتورةَ (كارين جوس) والدّكتورة (لويز ماون) في عام 2000 أداء خمس عملياتِ تَتضمّنُ قطع نافذة في غمدِ العصبِ البصريِ، لاختبار كفاءةِ تثقّيبِ غمدِ العصبِ البصريِ. هذه العملياتِ الثمانية أتت بما هو متوقّع بالنَتائِجِ ومتّسقة مع المعيارِ الروتينيِ للعنايةِ وبفائدة إضافية مِنْ جراحةِ الليزرِ والضرر الإضافي الأقل ما يمكنِ. أي مراجعة ليزر الإلكترون الحر للاستعمالات الطبيةِ تُسلّمُ الطبعةِ الأولى لتطبيقاتِ ليزرِ. منذ هذه النَتائِجِ الناجحةِ، كانت هناك عِدّة جُهود لبِناء الليزرِ السريريِ الصغيرِ (تيونابل - تنغيم) في مدى من الـ6 إلى 7 مايكرو متر بتركيبِ وطاقةِ النبضِة لإعْطاء اقل ما يمكن من الضرر الإضافي في النسيجِ الناعمِ. في الاجتماع السنوي عام 2006 للمجتمعِ الأمريكيِ للطبِّ وجراحةِ الليزرِ (أي إس إل إم إس)، ذَكرَ الدّكتور (روكس أندرسن) من مختبرِ (ويلمان) للتصوير الطبي بكليّة (هارفارد) الطبيّةِ ومستشفى مساتشوستس العام على التطبيقِ الطبيِ المحتملِ لليزرِ الإلكترون الحُرَّ في ذَوَبان الدهونِ بدون إيذاء جلدِ الخَنْق. وقد وُصِف أنّ في الأطوال الموجية للأشعة تحت الحمراءِ،سُخّنَ بالليزرِ ماء في نسيجِ، لكن في الأطوالِ الموجية المطابقة 915, 1210 و1720 نانومتر، تُسَخن الأسطح الدهنية الثانوية بشكل تفاضلي بقوة أكثر مِنْ الماءِ. التطبيقات المحتملة لهذا التحليل الحراري الضوئي الانتقائي (تسخن الأنسجة باستخدام الضوء) يَتضمّنُ الدمارَ الانتقائي لدهون الغدد الجلدية لمُعَالَجَة حبِّ الشباب، بالإضافة إلى الاستهداف الآخرِ للدهون المرتبطة بدهون الجسم بالإضافة إلى اللوحاتِ السمينةِ التي تُشكّلُ في الشرايينِ والتي يُمْكِنُ أَنْ تُساعدَ على مُعَالَجَة تصلب الشرايين وأمراض القلب.
. . . . . . . . . . . . . . . . . . . . . . . . . . . . . . . . . . . . . . . . . . . . . . . . . . . . . . . . . . . . . . . . . . . . . . . . . . . . . . . . . . . . . . . . . . . . . . . . . . . . . . . . . . . . . . . . . . . . . . . . . . . . . . . . . . . . . . . . . . . . . . . . . . . . . . . . . . . . . . . . . . . . . . . .
إزالة الدهون
Several small, clinical lasers tunable in the 6 to 7 micrometre range with pulse structure and energy to give minimal collateral damage in soft tissue have been created.[بحاجة لمصدر] At Vanderbilt, there exists a Raman shifted system pumped by an Alexandrite laser.[17]
Rox Anderson proposed the medical application of the free-electron laser in melting fats without harming the overlying skin.[18] At infrared wavelengths, water in tissue was heated by the laser, but at wavelengths corresponding to 915, 1210 and 1720 nm, subsurface lipids were differentially heated more strongly than water. The possible applications of this selective photothermolysis (heating tissues using light) include the selective destruction of sebum lipids to treat acne, as well as targeting other lipids associated with cellulite and body fat as well as fatty plaques that form in arteries which can help treat atherosclerosis and heart disease.[19]
التطبيقات العسكرية
تعتبر تقنية ليزر الإلكترون الحر (عند القوة البحرية الأمريكيةِ) كمرشّح جيد لسلاحِ طاقةِ مُوَجَّهِ ضدّ الصّواريخِ. التقدّم الهامُ في مستويات طاقة ليزر الإلكترون الحر تزايدت (اثبت (توماس جيفيرسن) أن معجّلِ ليزر الإلكترون الحر أكثر مِنْ 14 كيلو وات) ويَجِبُ أَنْ يَكُونَ من المحتملَ بِناء نوع متعدّدِ الميجاوات المضغوطَ من أسلحة ليزر الإلكترون الحر.وقد أعلن مكتب البحثِ البحريِ في 9 يونيو/حزيران 2009 بأنّ مَنحتْ (رايثيون) عقد لتَطوير 100 كيلو وات ليزر إلكترون حر تجريبي.
FEL prize winners
The FEL prize is given to a person who has contributed significantly to the advancement of the field of free-electron lasers. In addition, it gives the international FEL community the opportunity to recognize its members for their outstanding achievements.
- 1988 John Madey
- 1989 William Colson
- 1990 Todd Smith and Luis Elias
- 1991 Phillip Sprangle and Nikolai Vinokurov
- 1992 Robert Phillips
- 1993 Roger Warren
- 1994 Alberto Renieri and Giuseppe Dattoli
- 1995 Richard Pantell and George Bekefi
- 1996 Charles Brau
- 1997 Kwang-Je Kim
- 1998 John Walsh
- 1999 Claudio Pellegrini
- 2000 Stephen V. Benson, Eisuke J. Minehara, and George R. Neil
- 2001 Michel Billardon, Marie-Emmanuelle Couprie, and Jean-Michel Ortega
- 2002 H. Alan Schwettman and Alexander F.G. van der Meer
- 2003 Li-Hua Yu
- 2004 Vladimir Litvinenko and Hiroyuki Hama
- 2005 Avraham (Avi) Gover
- 2006 Evgueni Saldin and Jörg Rossbach
- 2007 Ilan Ben-Zvi and James Rosenzweig
- 2008 Samuel Krinsky
- 2009 David Dowell and Paul Emma
- 2010 Sven Reiche
- 2011 Tsumoru Shintake
- 2012 John Galayda
- 2013 Luca Giannessi and Young Uk Jeong
- 2014 Zhirong Huang and William Fawley
- 2015 Mikhail Yurkov and Evgeny Schneidmiller
- 2017 Bruce Carlsten, Dinh Nguyen and Richard Sheffield
- 2019 Enrico Allaria, Gennady Stupakov, and Alex Lumpkin
- 2022 Brian McNeil and Ying Wu
Young Scientist FEL Award
The Young Scientist FEL Award (or "Young Investigator FEL Prize") is intended to honor outstanding contributions to FEL science and technology from a person who is less than 35 years of age.
- 2008 Michael Röhrs
- 2009 Pavel Evtushenko
- 2010 Guillaume Lambert
- 2011 Marie Labat
- 2012 Daniel F. Ratner
- 2013 Dao Xiang
- 2014 Erik Hemsing
- 2015 Agostino Marinelli and Haixiao Deng
- 2017 Eugenio Ferrari and Eléonore Roussel
- 2019 Joe Duris and Chao Feng
- 2022 Zhen Zhang, Jiawei Yan, and Svitozar Serkez
انظر أيضاً
المراجع
- ^ Feldhaus, J.; Arthur, J.; Hastings, J. B. (2005). "X-ray free-electron lasers". Journal of Physics B. 38 (9): S799. Bibcode:2005JPhB...38S.799F. doi:10.1088/0953-4075/38/9/023. S2CID 14043530.
- ^ Huang, Z.; Kim, K.-J. (2007). "Review of x-ray free-electron laser theory". Physical Review Special Topics: Accelerators and Beams. 10 (3): 034801. Bibcode:2007PhRvS..10c4801H. doi:10.1103/PhysRevSTAB.10.034801.
- ^ Fain, B.; Milonni, P. W. (1987). "Classical stimulated emission". Journal of the Optical Society of America B. 4 (1): 78. Bibcode:1987JOSAB...4...78F. doi:10.1364/JOSAB.4.000078.
- ^ Benson, S.; Madey, J. M. J. (1984). "Quantum fluctuations in XUV free electron lasers". AIP Conference Proceedings. Vol. 118. pp. 173–182. doi:10.1063/1.34633.
- ^ Schöllkopf, Wieland; Gewinner, Sandy; Junkes, Heinz; Paarmann, Alexander; von Helden, Gert; Bluem, Hans P.; Todd, Alan M. M. (201). Biedron, Sandra G (ed.). "The new IR and THz FEL facility at the Fritz Haber Institute in Berlin". Advances in X-ray Free-Electron Lasers Instrumentation III. International Society for Optics and Photonics. 9512: 95121L. Bibcode:2015SPIE.9512E..1LS. doi:10.1117/12.2182284. hdl:11858/00-001M-0000-0027-13DB-1. S2CID 55435812.
- ^ "The FHI free-electron laser (FEL) facility". Fritz Haber Institute of the Max Planck Society (in الإنجليزية). Retrieved 2020-05-04.
- ^ Doerr, Allison (November 2018). "High-speed protein crystallography". Nature Methods. 15 (11): 855. doi:10.1038/s41592-018-0205-x. PMID 30377367.
- ^ Irving, Michael (2022-05-11). "World's most powerful X-ray laser now fires a million bursts per second". New Atlas (in الإنجليزية الأمريكية). Retrieved 2022-05-16.
- ^ "FERMI HomePage". Elettra.trieste.it. 2013-10-24. Retrieved 2014-02-17.
- ^ Doyuran, A., et al., Characterization of a High-Gain Harmonic-Generation Free- Electron Laser at Saturation. Phys Rev Lett, 2001. 86(26): p. 5902-5905.70.
- ^ I. Ben-Zvi, K.M. Yang and L.H. Yu, The ‘Fresh-Bunch’ Technique in FELs, Nuclear Instruments & Methods in Physics Research A318, 726 (1992)
- ^ S. Di Mitri, et al, Double Stage Seeded FEL with Fresh Bunch Injection Technique at FERMI@ELETTRA, Proceedings of IPAC2013, Shanghai, China, ISBN 978-3-95450-122-9 pp. 1185-1186
- ^ F. Sottocorona, et al., Phys. Rev. Accel. Beams 26, 090702 – Published 12 September 2023
- ^ Guanglei Wang et al, Millijoule Femtosecond X-Ray Pulses from an Efficient Fresh-Slice Multistage Free-Electron Laser, Physical Review Letters 132, 035002 (2024)
- ^ Amann, J.; Berg, W.; Blank, V.; Decker, F. -J.; Ding, Y.; Emma, P.; Feng, Y.; Frisch, J.; Fritz, D.; Hastings, J.; Huang, Z.; Krzywinski, J.; Lindberg, R.; Loos, H.; Lutman, A.; Nuhn, H. -D.; Ratner, D.; Rzepiela, J.; Shu, D.; Shvyd'ko, Y.; Spampinati, S.; Stoupin, S.; Terentyev, S.; Trakhtenberg, E.; Walz, D.; Welch, J.; Wu, J.; Zholents, A.; Zhu, D. (2012). "Demonstration of self-seeding in a hard-X-ray free-electron laser". Nature Photonics. 6 (10): 693. Bibcode:2012NaPho...6..693A. doi:10.1038/nphoton.2012.180. S2CID 122939995.
- ^ ""Self-seeding" promises to speed discoveries, add new scientific capabilities". SLAC National Accelerator Laboratory. August 13, 2012. Archived from the original on February 22, 2014. Retrieved 2013-11-06.
- ^ Jayasinghe, Aroshan; Ivanov, Borislav; Hutson, M. Shane (2009-03-18). "Efficiency and Plume Dynamics for Mid-IR Laser Ablation of Cornea". APS March Meeting Abstracts: T27.006. Bibcode:2009APS..MART27006J. Retrieved 2010-11-06.
- ^ "BBC health". BBC News. 2006-04-10. Retrieved 2007-12-21.
- ^ "Dr Rox Anderson treatment". Retrieved 2007-12-21.
للاستزادة
- Madey, John M. J. (1971). "Stimulated Emission of Bremsstrahlung in a Periodic Magnetic Field". Journal of Applied Physics. 42 (5): 1906–1913. Bibcode:1971JAP....42.1906M. doi:10.1063/1.1660466.
- Madey, John, Stimulated emission of radiation in periodically deflected electron beam, US Patent 38 22 410,1974
- Boscolo, I.; Brautti, G.; Clauser, T.; Stagno, V. (1979). "Free-electron lasers and masers on curved paths". Applied Physics. 19 (1): 47–51. Bibcode:1979ApPhy..19...47B. doi:10.1007/BF00900537. S2CID 121093465.
- Deacon, D. A. G.; Elias, L. R.; Madey, J. M. J.; Ramian, G. J.; Schwettman, H. A.; Smith, T. I. (1977). "First Operation of a Free-Electron Laser". Physical Review Letters. 38 (16): 892–894. Bibcode:1977PhRvL..38..892D. doi:10.1103/physrevlett.38.892.
- Elias, Luis R.; Fairbank, William M.; Madey, John M. J.; Schwettman, H. Alan; Smith, Todd I. (1976). "Observation of Stimulated Emission of Radiation by Relativistic Electrons in a Spatially Periodic Transverse Magnetic Field". Physical Review Letters. 36 (13): 717–720. Bibcode:1976PhRvL..36..717E. doi:10.1103/physrevlett.36.717.
- Gover, Avraham; Livni, Zohar (1978). "Operation regimes of Cerenkov-Smith-Purcell free electron lasers and T.W. Amplifiers". Optics Communications. 26 (3): 375–380. Bibcode:1978OptCo..26..375G. doi:10.1016/0030-4018(78)90226-2.
- Gover, A.; Yariv, A. (1978). "Collective and single-electron interactions of electron beams with electromagnetic waves, and free-electron lasers". Applied Physics. 16 (2): 121–138. Bibcode:1978ApPhy..16..121G. doi:10.1007/bf00930376. S2CID 18497302.
- "The FEL Program at Jefferson Lab" Jefferson Lab Free-Electron Laser Program
- Brau, C. A. (1988). "Free-Electron Lasers". Science. 239 (4844): 1115–1121. Bibcode:1988Sci...239.1115B. doi:10.1126/science.239.4844.1115. PMID 17791971. S2CID 45638507.
- Paolo Luchini, Hans Motz, Undulators and Free-electron Lasers, Oxford University Press, 1990.
. . . . . . . . . . . . . . . . . . . . . . . . . . . . . . . . . . . . . . . . . . . . . . . . . . . . . . . . . . . . . . . . . . . . . . . . . . . . . . . . . . . . . . . . . . . . . . . . . . . . . . . . . . . . . . . . . . . . . . . . . . . . . . . . . . . . . . . . . . . . . . . . . . . . . . . . . . . . . . . . . . . . . . . .
وصلات خارجية
- Chapter dedicated to XFEL, included in the web to learn crystallography CSIC
- Lightsources.org
- LCLS the Linac Coherent Light Source, the world's first hard x-ray FEL at the SLAC National Accelerator Laboratory
- FERMI, the new FEL at the ELETTRA synchrotron in Trieste
- Free-Electron Laser Open Book (National Academies Press) Archived 2008-07-19 at the Wayback Machine
- The World Wide Web Virtual Library: Free-Electron Laser research and applications
- European XFEL
- PSI SwissFEL
- SPring-8 Compact SASE Source
- PAL-XFEL, South Korea
- Electron beam transport system and diagnostics of the Dresden FEL
- The Free Electron Laser for Infrared eXperiments FELIX
- W. M. Keck Free Electron Laser Center
- Jefferson Lab's Free-Electron Laser Program
- Free-Electron Lasers: The Next Generation by Davide Castelvecchi New Scientist, January 21, 2006
- Airborne megawatt class free-electron laser for defense and security
- FERMI@Elettra Free-Electron Laser Project
- Center for Free-Electron Laser Science (CFEL)
- FELIX Laboratory, free-electron lasers in Nijmegen, the Netherlands